For centuries, the building envelope was thought of as not only providing an exterior “look” to a building but also as an integral component of its support structure.
Just think of the pyramids — huge structures assembled stone by giant stone, said Connell Miller, an engineering PhD candidate at Western University in London.
Then, in the mid-1800s, the British construction industry began to challenge this notion, most notably in the development of the Crystal Palace in the 1850s in Hyde Park, London where glass enclosed a cast iron structural framework.
"All (the glass) is doing is protecting (the structure) from the elements," Miller said.
The advent of the skyscraper in Chicago later that same century would further drive a separation between a building’s exterior and its structure until it crystallized in our present-day understanding of cladding as an exterior "skin" that protects a building from elements such as wind, rain and snow. But with the use of structural steel to create taller and taller buildings came another challenge: how to ensure these structures and their new non-weight-bearing cladding could resist the effects of the elements and, in particular wind?
The answer to this question would become the life’s work of a professor at a fledgling engineering school in London, Ont. The solutions he found would have a fundamental impact on building and bridge construction here in Canada and around the world.
Alan Davenport knew from an early age how much impact wind could have on buildings. The pioneer in wind engineering in Western’s Faculty of Engineering had been born in 1932 in Madras, India (now Chennai, capital of Tamil Nadu, an Indian state) where tropical storm gusts during monsoon season necessitated the use of sandbags to protect the family home.
Davenport, who went on to study mechanical sciences and engineering in Britain and Toronto, was also a pilot in the Royal Canadian Navy. At that time of his graduate studies, the standard approach to determining wind’s effects on buildings was much the same as that used for airplanes.
"You’d read the wind speed at the airport and then recreate that wind speed in a wind tunnel, stick your building in there and see how it reacted," said Randy Van Straaten, another PhD student at Western.
From his own experiences, however, Davenport knew that wind performs differently at different levels from the ground. Even just a few feet off ground on a roof or the top of a silo it’s much windier; meanwhile, closer to the surface, objects such as other buildings or unusual land features cause wind to start rolling and create turbulence, Van Straaten said.
When Davenport was recruited to Western University in 1961, he put his theories into action by replicating these boundary layer wind effects in a unique wind tunnel that had been expanded at one end. He added obstacles to make it windier farther from the surface and also added "fake" buildings on the ground level to create turbulence.
During the 1960s, he honed the testing approach on the World Trade Centre in New York building design. Had the building been designed according to the wind testing standards of the time, "they never would have built it," Van Straaten said.
"They needed more accurate modelling — also to capture the sheltering of all the buildings around it."
During the 1970s plans for most of the world’s tallest buildings were analyzed and tested in the wind laboratory that Davenport developed, including the CN Tower which, when it opened in 1976, was declared the world’s tallest free-standing structure.
Since then, Western has continued to play a key role in assessing the impact of wind on structures, developing in 2008 the Insurance Research Lab for Better Homes (Three Little Pigs Lab) that would allow the assessment of wind loads on full scale houses and light-frame structures and, in 2011, the Wind Engineering, Energy and Environmental Research Institute, the world’s first three-dimensional testing chamber.
Exteriors remain a focus. Miller, for instance, is studying how best to create standards for wind’s impact on vinyl siding. The popular material for residential dwellings reflects the "one or two leading insurance costs on buildings, second only to shingles" when it comes to wind damage, he noted.
Most current wind resistance design standards used for vinyl cladding are not accurate because they are derived from numbers based on a uniform wind pressure, Miller explained. Wind, however, rarely applies uniform pressure, and the pressure experienced on the exterior of vinyl cladding is different from that experienced on its interior. "So what we’re trying to do is do is do experiments to prove this is what is actually happening; these are the real physics of how the wind is transferred from the outside of the cladding system to the inside of it, and this is why the standards are inaccurate," he said.
The goal is to come up with more accurate standards.
Miller’s work may lead to a method of developing standards that could be applied to other types of cladding as well, said Van Straaten.
"The science is advancing on that quite a bit."
Such work becomes even more crucial as the industry focuses on the effects of climate change.
Current techniques used to estimate design standards for wind, rain and snow loads as well as others, "essentially assume that things are static," Van Straaten explains.
But if the climate is changing and wind speed means, for instance, are rising, "the methods we’ve been applying, they don’t really hold on a statistical level."
The industry’s growing emphasis on creating energy efficient buildings may well spark a rethink of cladding’s role in a structure and the materials used as well, he suggests.
For instance, LEED pilot credits for building resilience include a credit for thermal resilience. The credit sets standards for how much a building would overheat if electricity is lost in the summer or how much it would cool of electricity and gas were lost in the winter.
Those working on a revision of the Toronto Green Standard for mid to high-rise residential and non-residential development have shown some interest in adding this credit, Van Straaten noted.
Had it not been for the innovations of engineers like Davenport, however, taking such an analytical approach a building’s response to the elements — and cladding’s role in the process — would be all that much more difficult to do today.
"He was the best wind engineer in the world, working on some of the top landmarks worldwide," said Hesham El Naggar, Western University engineering associate dean, research and external services, was quoted in a university article remembering Davenport’s groundbreaking career.
Davenport died in 2009.
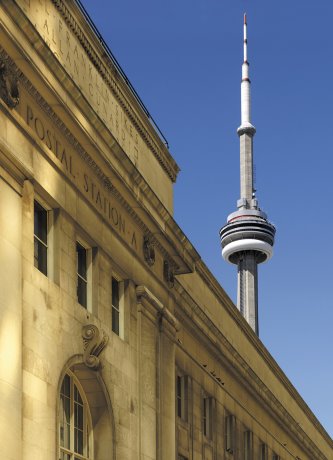
1/2
The wind laboratory at Western University was key to understanding the impact of wind on the CN Tower.
Photo: TOM ARBAN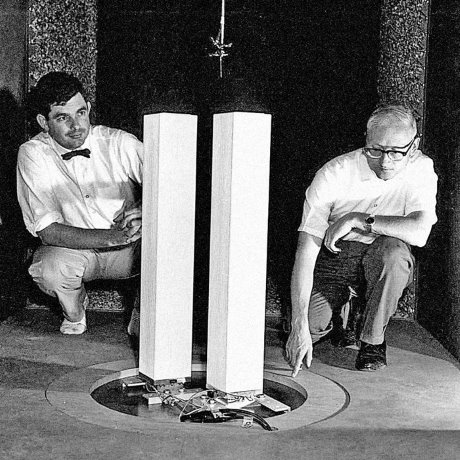
2/2
Alan Davenport
Recent Comments
comments for this post are closed